3. The animal LCA likely possessed many different cell types
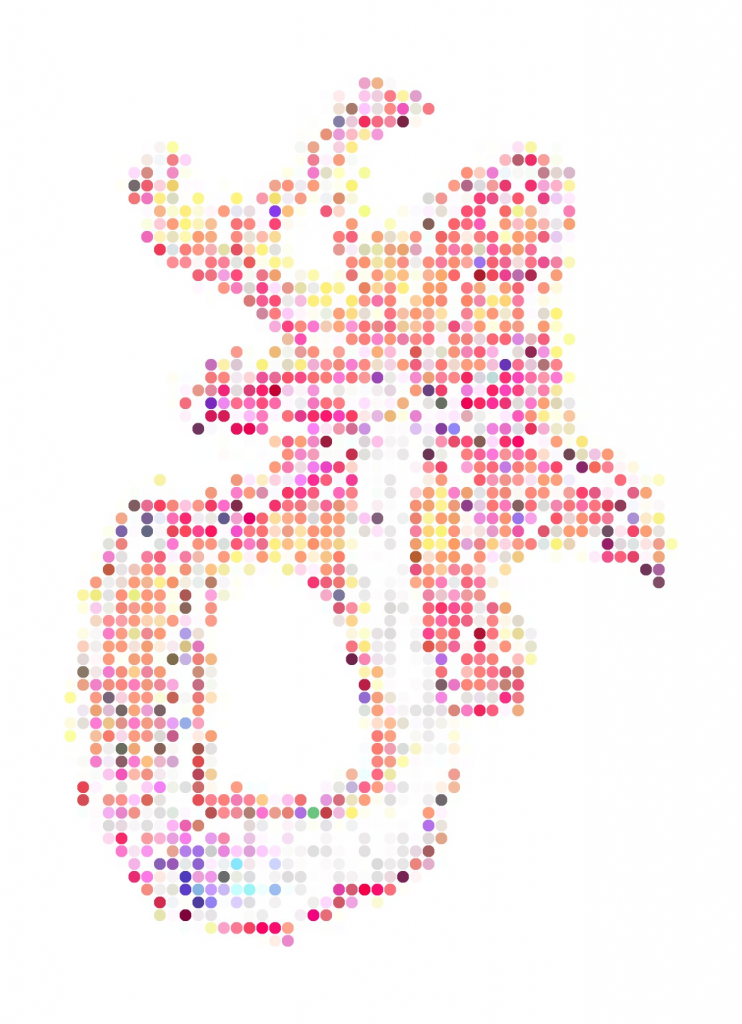
A major feature of animals is the spatial distribution of labour between coexisting cells within a single multicellular entity. The building blocks of multicellular bodies are different kinds of cell types, each specialized in different roles within the whole organism. Cell types have their own sets of expressed genes used in different processes (e.g., contraction, secretion, signaling and reception), that are normally regulated by well-defined genetic programs (a set of TFs and other specific regulatory mechanisms). This implies that some genes are expressed by certain cell types but not others – in other words, each cell type expresses a limited number of genes encoded in the genome. On the other hand, the totality of genes in the genome of a unicellular organism may be used by a single cell throughout its life. The genome partitioning into functional modules accessed by different cell types reflects an increase in regulatory mechanisms to determine diverse cell fate genetic programs (Arendt et al., 2019). Understanding the origin of animals, therefore, requires asking questions about the evolutionary origins of cell types, and their mechanisms of differentiation.
Comparative genomics provides information only about the presence or absence of certain genes in the organism. Therefore, sequencing alone provides limited information on how those genes are expressed and regulated in different cells, and on the putative identities of the cells within an organism. Insights in this field have come hand in hand with advances in single-cell genomics (Hwan, Lee and Bang, 2018; Stuart and Satija, 2019; Sebé-Pedrós et al., 2018a, 2018b). It has been reported that cell-type diversity increases within animals at each step of body plan complexification, with relatively low cell type diversity in early-branching animals contrasting with the hundreds or thousands of cell types in bilaterians and in vertebrates, respectively. However, new research is showing that cell type diversity is more complex and varied in animals than we thought.
Porifera and Cnidaria showcase cryptic cell type complexity at the molecular and the regulatory level
Many of the bona fide cell types of non-bilaterians have been classically described at the morphological level, but molecular data have allowed the definition of numerous identities previously unnoticed (Marioni and Arendt, 2017). Cell atlases of sponges and cnidarians (Musser et al., 2019; Sebé-Pedrós et al., 2018b; Siebert et al., 2019) have allowed the identification of transcriptional signatures in the form of correlated and differential gene expression across the transcriptomes of different cells, leading to the definition of numerous “metacells” in these organisms (Baran et al., 2019; Sebé-Pedrós et al., 2018b)*. Based on their expression profiles, these metacells can be linked to putative functions, such as muscle or cnidocyte cells in Hydra and the starlet sea anemone Nematostella, or archaeocytes in sponges (Musser et al., 2019; Sebé-Pedrós et al., 2018b; Siebert et al., 2019; Sogabe et al., 2019), or can be used to detect novel, undiscovered cell types, such as nitric oxide-sensitive contractile cells or neuroid-amoeboid cells in Spongilla (Musser et al., 2019).
* Metacell: In single-cell genomics, a subgroup of homogeneous scRNASeq profiles with only local variance relative to the total dataset, useful for clustering and quantitative gene expression analyses. Ultimately, it can be related to certain cell types, but only upon experimental validation.
One main recognisable signature of these cell types is the expression of TFs: there are hundreds of them differentially expressed in Nematostella; 85% of these hundreds are of metazoan origin and half are specific to certain metacells. These numbers reflect the importance of TFs diversification in the lineage that led to animals (de Mendoza et al., 2013). Correlation of gene expression between TFs suggests there exists a hierarchy of control to define the identity of the cells (Sebé-Pedrós et al., 2018b), meaning the control of cell-type identity emerges from the control at the tissue level, from a broader to a more specific fate. Distal regulation may also play a role in the specification of cell types, as many of the cell-type-specific genes have associated putative enhancers spanning multiple kilobases upstream (Sebé-Pedrós et al., 2018b). Thus, animal cell type diversity can be defined and stabilized by specific regulatory programs (i.e., networks of TFs per cell type and other regulatory control mechanisms), linking organismal complexity to genome regulatory complexity, which may be required for more diverse cell type repertoires (Sebé-Pedrós et al., 2018b).
An especially large number of cell types correspond to neuronal identity in these species (Sebé-Pedrós et al., 2018b). These cells show extensive conservation of neuronal effector gene repertoires with other animal species, but not at the level of coexpression, supporting the existence of an ancestral cell type with neuronal characteristics, at least at the onset of cnidarians and bilaterians. Interestingly, a group of Spongilla cells named neuroids surround other cells and express a combination of TFs and effector genes related to immunity and synaptic communication (Musser et al., 2019). Animals like Nematostella, Hydra and Spongilla have thus been able to use basal regulatory machinery and different ancestral pathways to evolve their own types of cells. This fact reinforces the possibility of an animal LCA machinery related to that of nerve and immune cells which could have later diverged independently in each lineage, giving rise to similar structures, such as the nervous system.
Integrating single cell information from different lineages
So far, single-cell approaches are bringing a much deeper insight into cell type differentiation in many early-branching Metazoa by improving taxon sampling – adding sponges, comb jellies and placozoans to the comparisons – and by revealing cryptic cell type diversity (Sebé-Pedrós et al., 2018a). These analyses have revealed how sponge larvae and adults exhibit different cell types, an unexpectedly high diversity of cell types in ctenophores, and the presence of peptidergic cell types in placozoans (Sebé-Pedrós et al., 2018a).
Interestingly, these species show differences in the control of cell type-specific gene expression, such as a large diversity of TFs and very specific promoter motifs, versus the use of distal elements (Sebé-Pedrós et al., 2018a). Because of these differences in very different lineages of early branching animals, it is likely that cell type genome regulation in the animal LCA involved a heterogeneous program of distal regulation and complex TF networks. However, the large evolutionary distances and high divergence of these lineages from the ancestor makes it difficult to find homologous cell types between them. Only a limited number of essential features, such as the expression profile of epithelial or ciliated cells, are conserved across species. Moreover, most of the genes expressed in all cell types in these species are either found in all eukaryotes or are lineage-specific, and in all cases, it appears that novel genes are more limited to specific cell types (Sebé-Pedrós et al., 2018a).
From this scenario of poor conservation and high divergence, we can make three observations:
- First, the very limited living record of early branching animals becomes evident. The fossil record shows that many animal groups present in the Cambrian explosion went extinct, leaving no representatives to this day, a situation with little prospect of change due to the discovery of only three animal phyla in the past thirty years (Kristensen, 2002), and with all the documented metazoan diversity by environmental metabarcoding belonging to already existing phyla (López-Escardó et al., 2018).
- Second, the currently extant early-branching animals are very derived and specialized, suggesting that secondary losses and simplification fostered the evolution of new structures and genes in these lineages (O’Malley et al., 2016).
- Third, while tracing the origin of specific cell types to the origin of Metazoa proves a difficult task, these approaches show that animals have been able to diversify and specify the fates of their cells in many different ways but using the same mechanisms of genomic regulation and the same effector machinery responsible for cell physiology.
Thus, we can conclude that the animal LCA was already rich in cell types which share some of their cellular foundations with those in extant species. Future integration of all the newly developed single-cell techniques in more non-model animal species will help to uncover even more aspects of these foundations (Stuart and Satija, 2019).
The next logical question we must ask is, therefore, how does all of this genome and diversity of cell types translates into the diversity of features and body plans observed in animals. In the next entry, we will frame these and more ideas together to have a better understanding of the life history of the animal LCA. We will dive a bit more into the geological and ecological contexts, and we will discuss some more integrative aspects that might explain the evolutionary plasticity of the animal LCA.